Current Research Projects
in the Casadonte Group
Chemical Applications of Ultrasound
Sonochemistry (the use of low- and high- intensity ultrasound to drive chemical reactions) has become a significant research field over the past thirty years. When ultrasound is transmitted through a liquid, high-energy species are produced through acoustic cavitation, i.e., the generation, growth, and nearly adiabatic implosion of vacuoles in solutions. The short collapse lifetimes (< 1 μs) and high dynamic pressures (> 100 atm) generated during transcient cavitation are largely responsible for the extreme temperatures (ranging from 800 - 17,000 K or even higher), enormous momentum transfers, and rapid quenching rates observed upon bubble collapse. We can use these physical extrema to facilitate a variety of different types of reactions with practical applications.
The Sonochemical Production of Biodiesel Fuel Using Solid Lewis-Acid Catalysts
(Shahid Islam)
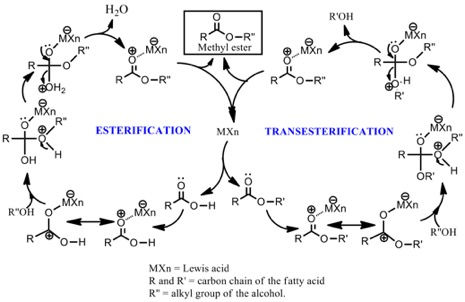
I have been working in the Casadonte Research group since I joined Texas Tech University to pursue a Ph.D. degree. My research is entitled "Ultrasound-assisted solid Lewis acid-catalyzed transesterification of Lesquerella fendleri oil to biodiesel, an alternate to petroleum diesel. In order to replace of traditionally used but environmentally corrosive and expensive catalyst systems, I has been using metal-based solid Lewis acid catalysts that provide more sustainable, easy-separation, and green synthesis approach with reusability and more catalytic activity.
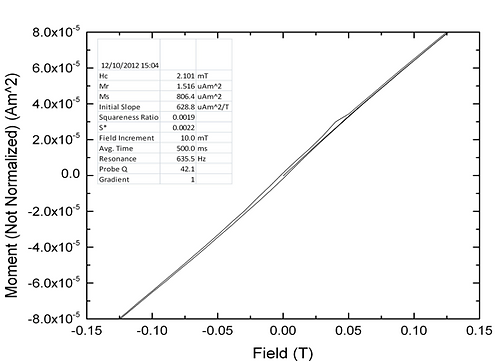

Materials Sonochemistry
(Ashley Moreno-Gongora)
My research focuses on two main areas: 1) effects of varying the ultrasonic frequency on nanomaterials 2) sonochemical preparation of magnetic nanomaterials with an emphasis on lanthanide ferrites.
1) Ultrasound (waves occurring at or above 20 kHz) can be introduced into an appropriate vessel via an ultrasonic horn to generate microbubbles that oscillate at the transducer’s resonate frequency. Once the bubbles reach a critical diameter, adiabatic collapse occurs producing temperatures and pressures of ~5000 K and ~1000 atm respectively, also known as acoustic cavitation. The resulting environment is favorable for physical and chemical reactions to take place.
Sonicators are devices that distribute ultrasonic waves into a vessel and there are three main types used in our lab. Firstly, the cleaning bath delivers low-intensity ultrasound and is typically used for agitating materials and the production of micron-sized particles. Secondly, the direct immersion sonicator introduces high-intensity ultrasound into the reaction with the ability to chemically and physically bond metals together. The third and most unique device is the heterodyne sonicator which uses two different frequencies resulting in a net frequency equal to the difference of the two original waves known as the heterodyne effect. My research attempts to observe the effects of ultrasound on nanomaterials primarily using the heterodyne sonicator.
2) Spinel ferrites have gained attention in the past decade due to their ability to be influenced by an external magnetic field. Such properties are necessary for hyperthermia treatments, a promising type of cancer therapy that allows for localized heating to tumor sites using magnetic nanoparticles. Since the premise of the technique hinges on magnetic materials, I attempt to use lanthanide spinel ferrites as the basis of my synthesis. Lanthanides, atomic numbers 57-71, are characterized by their considerable magnetic moments due to the large number of unpaired electrons distributed in the seven 4f orbitals. Ferrites are well-established permanent magnets used in a variety of different application, especially magnetic electrical components. My goal is sonochemically synthesize lanthanide ferrites desirable for hyperthermia therapy by combining the two magnetically favorable components.
Hydrogen Storage
(Lucy Kim)
My research is mainly concerned with nanomaterials and hydrogen storage in those materials. The carbon nanomaterials have been extensively studied in various fields. Hydrogen has been introduced as a possible next-generation fuel. However, there are lots of challenges which prevent it from being used as an everyday fuel source. One of the biggest challenges is the storage of the hydrogen in a fuel cell. The highly symmetric fullerenes show the potential in the presence of ultrasound and a catalyst as a superior gas-storage system. We are working to optimize the catalytic process (both storage and release of hydrogen) using low-intensity ultrasound.
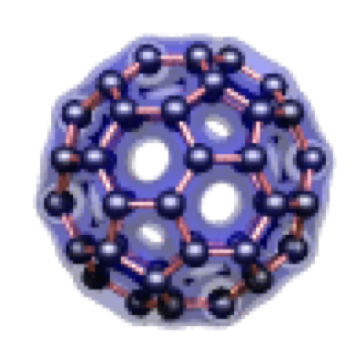
Ultrasound-Activated Catalysis
(Hannah Harbin)

We collaborate closely with Dr. Casadonte and his group on the research of catalyst assisted hydrogen storage in nano materials. Using low intensity sonication and a catalyst composed of nickel metal supported on silica, we hydrogenate carbon nanomaterials. Our catalyst is prepared via incipient wetness impregnation and calcination to produce 10 wt% of Ni to SiO2, where the nickel metal is believed to be the active site of the reaction. Initially, Ni(NO3)2 and SiO2 is dissolved in water and stirred to produce Ni(NO3)2/SiO2. This is then calcined in air to produce NiO/SiO2. Finally, this is calcined in UHP H2 to reduce the catalyst to Ni/SiO2, which is our desired nickel metal active site needed to store hydrogen in our nano materials.
Sonochemical Environmental Remediation Using Nanoferrites
(Lindsey Bishop)
My research was conducted to explore the use of sonication to synthesize ferrite and substituted ferrite (MFe2O4) nanoparticles with catalytic properties. One specific application of these catalysts would be to increase the reaction rate for the degradation of acid orange. Acid Orange is a common colorant that is dumped into the China Sea as industrial waste, causing algae blooms. Acid Orange can be oxidized to remove the orange dye efficiently without causing more harm to the environment. A variety of metal ferrites that could potentially be used as catalysts in this reaction were produced by combining stoichiometric ratios of the desired metal salt and ferric chloride hexahydrate. This solution was sonicated in a direct immersion sonicating bath for one hour. The resulting solution was treated with a base until a precipitate formed. This precipitate was collected and dried. The catalytic abilities of the more than a dozen metal ferrites have been tested in a timed oxidation reaction, and the results correlated with the nature of the metals and the resulting structures.
MSO4 • nH2O + Fe2(SO4)3 • nH2O --)))----->
Set pH = 9.0 (NaOH) -------> 2M0.5Fe(O)(OH)•H2O + 4 Na2SO4
2 M0.5Fe(O)(OH)•H2O + 1/2O2 ------------------------------> MFe2O4 + 3 H2O
Calcine (300 °C; 1 hr)
Cu(I) Phenanthroline Photochemistry
Our group has been working in the area of Cu(I) phenanthroline chemistry for over 30 years. We have modified every position on 1,10-phenanthroline in order to develop ligand sets that will generate metallopolymers and supramolecular complexes which are fully conjugated. The ultimate goal of the research, besides the development of novel 1-, 2-, and 3-dimensional supramolecular architectures, is to photoelectric circuit devices.
The compounds on the left contain 1,10-phenanthroline and 1,3,5-triza-7-phosphaadamantane (PTA). This is a water-soluble phosphine which allows us to generate water stable Cu(I) complexes with intense luminescence due to a Metal-to-Ligand charge transfer (MLCT) state. The PTA has the ability to act as a monodentate ligand as well as form a metallopolymer with alternating phosphorous-nitrogen bonds to the copper(I) centers, as shown in the crystal structure below,
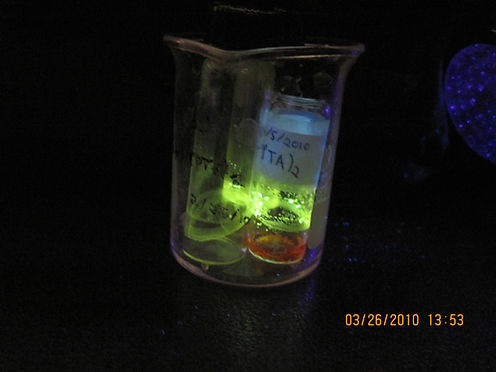
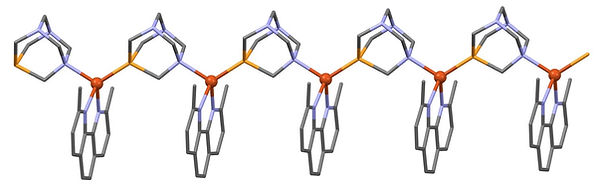dmp(PTA)%20Polymer%20copy.jpg)
'm a paragraph. Click here to add your own text and edit me. It’s easy. Just click “Edit Text” or double click me to add your own content and make changes to the font.

We have also been preparing compounds using two of the other isomers of phenanthroline, 1,7- and 4,7-phenanthroline. We have found with these ligands that once one of the nitrogens from the phenanthroline binds to a metal center, it deactivates the other nitrogen from binding. We have names ligands of these type "inhibited" ligands. The non-chelating ligands have medicinal uses as bacteriocidal agents. We are currently developing water-soluble 1,7- and 4,7- phenanthrolines complexes containing PTA, as in the compound shown to the left, which can be used to destroy biofilms.